Home Page
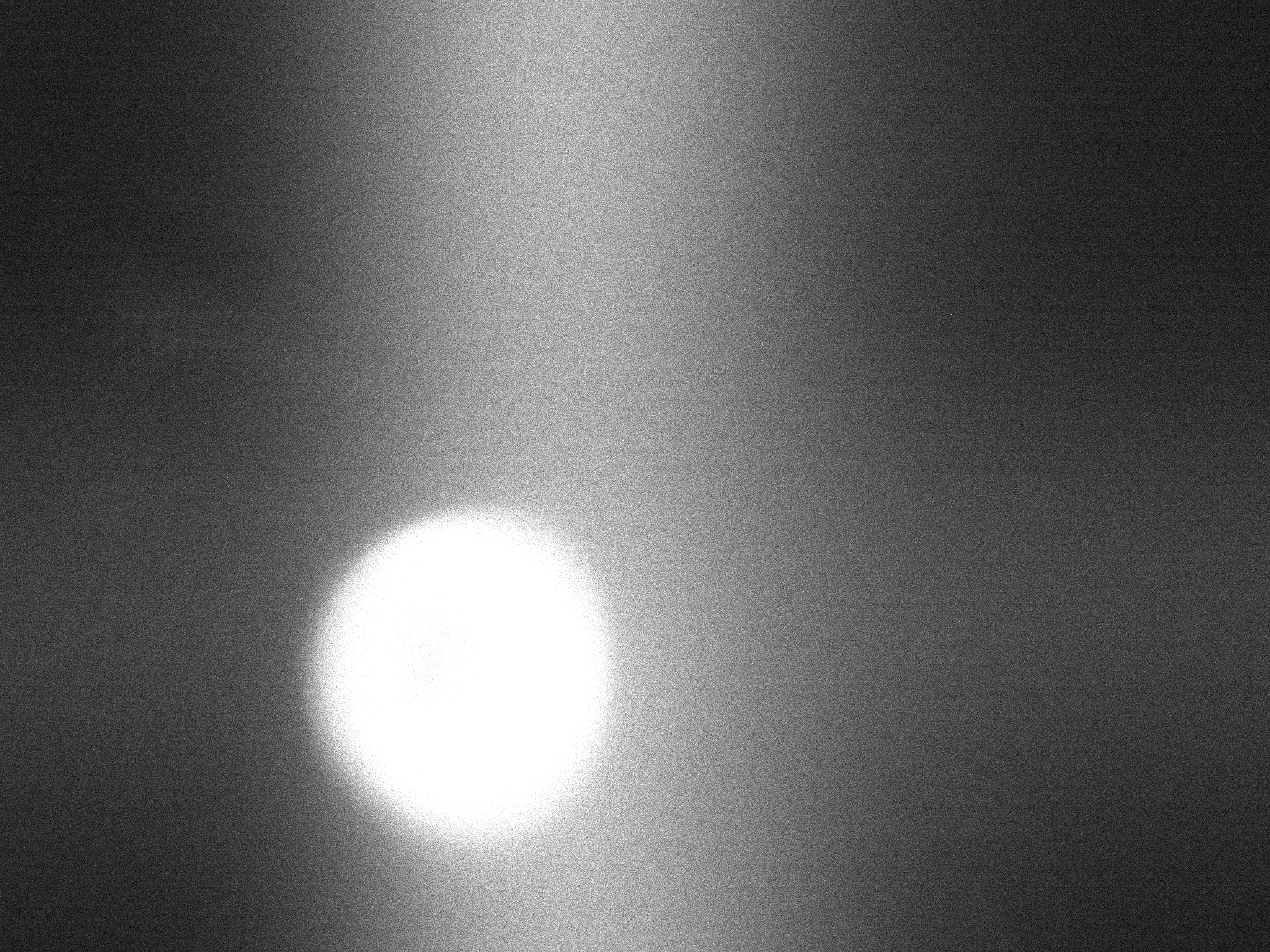
The first ultra cold atom in National Central University
At Comblaser LAB S4-415, 2023 05 / 22 AM 6:49
"Comb laser, as one of the most sexy light sources in comtempary, simutenously yields high resolution in femtosecond scale and optical clock level, dual advantages of wideband and high coherence, since that a new door was opened for us to play in the beautiful garden of science"
Welcome
About us
Our laboratory is in the field of so-called AMO (atomic, molecular and optical science), especially focus on high-precision measurement. We built up our own laser system for laser stabilization to atomic transition, that is the reason that students in our laboratory in general know about laser physics and electronics. Judgeing from our recent publications, you can find that we are able to offset lock laser frequency to sub-mini Hz level and we are able to build up secondary optical clock. Comb laser technology, which was appreciated by the Nobel committee at 2005, is the unique feature of our laboratory in Taiwan. Recently we collaborate ith Japan gravitational wave observatory (Kagra) for a project of “photon calibrator”, by using comb laser technology.
Laser System
M.S: 余欣鴻(Hsin-Hung Yu)、Undergraduate Students:鄧宇辰
This thesis attempts to develop a offset-frequency locking technology for the laboratory, which we call pulse offset frequency locking technique, so that ordinary mode-locked lasers can also indirectly refer to cesium atomic clocks through an optical comb laser. The key It is to use an optical comb laser with a self-reference system as a frequency reference for other mode-locked lasers that cannot confirm their own carrier-envelope offset frequency. This technique is mainly used to study the direct frequency-comb spectroscopy of Cs-Rb mixed atoms, mainly to study Cs:6S1/2 → 6P3/2 → 8S1/2 and Rb:5S1/2 → 5P3/2 → 5D5/2 stepwise two-photon transition. Among them, the Rb spectral line is one of the frequency standards suggested by BIPM. This research helps to develop experimental techniques for building optical clocks using comb lasers and Cs-Rb mixed atomic cell. In the past, the mode-locked laser used in the laboratory needed to rely on the beat frequency with other stabilized single-frequency lasers to obtain the carrierenvelope offset frequency. However, our stabilized single-frequency laser cannot directly give the absolute frequency.At that time, the senior of the laboratory found that the Cs spectra could have an error of 200∼300kHz compared with the theoretical calculation. In order to make up for the defect that the absolute frequency cannot be directly given in the experiment, I locked the mode-locked laser with the pulse offset frequency locking technique, and used this laser to scan the atomic spectral lines. We confirmed the error of 300kHz again and found that the reason is the Zeeman shift caused by the magnetic field on the atomic cell. We try to correct such errors and temporarily verify the reliability of the pulse offset frequency locking technique for scanning atomic spectra . According to the past experimental experience of Cs:6S1/2 → 8S1/2 direct twophoton transition, We think that the 6S1/2 → 8S1/2 two-photon transition of Cs is insensitive to magnetic fields. However, through this experiment we found that if it is a stepped two-photon transition, it may be very sensitive to magnetic fields.
In this paper I will explain the difference between the two types of two-photon transition from basic atomic physics.
Keywords: Optical frequency comb, Offset-frequency locking, Pulse synchronization, Direct frequency-comb spectroscopy.
https://hdl.handle.net/11296/44wue2
https://comblaser.phy.ncu.edu.tw/index.php/comblaser-brief-english/
M.S:石宇哲(SHIH, YU-JHE)、祝麒翔(Chi-Hsiang Chu)、Undergraduate Students:林宜廷
This project is an optical clock project that we cooperated with Chunghwa Telecom Laboratories.
In this thesis, we have measured the clock transition of rubidium. we first developed the differential Zeeman shift by Helmholtz coil, which coefficient of 87Rb 5S1/2(F = 2) → 5D5/2(F = 4) is −48.9 ± 2.6 kHz/G. After that, we have measured the Rb self-collision shift in a vacuum chamber system, and the linear coefficient is −120±70 Hz/µTorr. On the other hand, For the stability of the frequency-stabilized laser, the Allan deviation can reach 3×10( − 13) within 1000 second of integration time. Finally, we have measured the rubidium 5S1/2(F = 3) → 5D5/2(F ′ = 1 ∼ 5) transition, and the results are only ±5 kHz difference with the suggested value of Certificate in Investment Performance Measurement(CIPM).
https://hdl.handle.net/11296/842d4d
Postdoc:(Taro mashimo)真下太郎、PH.D:陳伯煒 、Undergraduate Student:朱致頡
Repumping laser For creatig the cold cesium atoms, the cooling laser frequency is tuned to D2 line (from ground state F=4 to excited state F’=5). There is a possibility of transition from F=4 to F’=4 and decay to F=3 state, which causes the depopulation of F=4 state. In order to prervent such situation, we use an additional laser called repumping laser. This laser has the resonance from ground state F=3 to excited state F’=4 and pumps the atoms in F=3 state back to cooling cycle.
Ph.D:陳可翰(Ko-Han Chen)、M.S:張峻堯(Chun-Yu Chang)
Nowadays, the photon calibrators are used to be the main calibrator of the gravitational wave
interferometers in LIGO, VIRGO and KAGRA. The photon calibrators connect the relationship between the radiation pressure and the displacement of the interferometer mirror. The displacement give us the information of gravitational wave, e.g., the distance and the orientation. However, the laser power measurements among international experimental results show the discrepencies.
Due to the inevitable aging and the inconsistent quantum efficiency of the optical-power sensitive elements, laser photon detectors can’t serve as absolute power standards. Here we propose that power measurement can be referenced to atomic
spectrum. By precise measuring AC-Stark effect in cesium two-photon absorption system, laser intensity change can be treated as a digitized value via transition frequency shifts. In the meantime, we construct a high-finesse cavity which can define the beamshape well inside. Thus, we can calculate the atom-photon interaction range and translate the laser intensity to the exact power.
https://comblaser.phy.ncu.edu.tw/index.php/2022/04/28/khan_expriment_profile/
PH.D:吳建明(Chien-Ming Wu)、劉子維(Tzu-Wei Liu)、陳可翰(Ko-Han Chen) M.S: 吳淑蓉(Shu-Rong Wu)、李奇 (Chi Lee)
We present an alternative scheme for determining the frequencies of cesium (Cs) atom 6S–8S Doppler-free transitions. With the use of a single electro-optical crystal, we simultaneously narrow the laser linewidth, lock the laser frequency, and resolve a narrow spectrum point by point. The error budget for this scheme is presented, and we prove that the transition frequency obtained from the Cs cell at room temperature and with one-layer μ-metal shielding is already very near that for the condition of zero collision and zero magnetic field. We point out that a sophisticated linewidth measurement could be a good guidance for choosing a suitable Cs cell for better frequency accuracy.
https://comblaser.phy.ncu.edu.tw/index.php/822nm/
1. C. M. Wu, T. W. Liu, M. H. Wu, R. K. Lee, and W. Y. Cheng, “Absolute frequency of cesium 6S-8S 822-nm two-photon transition by a high-resolution scheme”, Opt. Lett. 38, 3186 (2013)
2. C. M. Wu, T. W. Liu, and W. Y. Cheng, “Quantum interference in two-photon spectroscopy for laser stabilization and cesium cells comparison“, Phys. Rev. A 92, 042504 (2015)
3. K. H. Chen, C. M. Wu, S. R. Wu, H. H. Yu, T. W. Liu, and W. Y. Cheng, “Influence of atmospheric Helium on secondary clocks“, Opt. Lett. 394464
Contant us
(phone) : (03)4227151 ext 65308
Professor Email : wycheng@ncu.edu.tw